[ad_1]
Alone Tyrannosaurus rex sniffs the humid Cretaceous air, scenting a herd of Triceratops grazing beyond the tree line. As the predator scans the floodplain, its vision suddenly snaps into focus. A single Triceratops has broken off from the herd and wandered within striking distance. Standing motionless, the T. rex formulates a plan of attack, anticipating the precise angle at which it must intersect its target before the Triceratops can regain the safety of the herd. The afternoon silence is shattered as the predator crashes though the low branches at the edge of the forest in hot pursuit.
T. rex has hunted Triceratops in so many books, games and movies that the encounter has become a cliché. But did a scene like this one ever unfold in real life? Would T. rex identify its prey by vision or by smell? Would the Triceratops be warned by a loudly cracking branch or remain oblivious because it was unable to locate the source of the sound? Could T. rex plan its attack like a cat, or would it lash out indiscriminately like a shark?
Ever since dinosaurs were first described in the early 1800s, paleontologists have debated their intelligence, sensory capabilities and behavioral complexity. Early investigations relied on natural endocasts, which are casts formed when sediment fills the empty space in a skull. These casts replicate the shape of the braincase’s contents in life. The conventional wisdom long held that all dinosaurs had tiny brains and therefore unsophisticated behaviors. Perhaps the most amusing example of this view of dinosaur intelligence came from 19th-century paleontologist Othniel Charles Marsh, who hypothesized that the armored dinosaur Stegosaurus had a second brain near its rump to supplement the walnut-size brain in its skull. This idea was based on a vaguely braincase-shaped expansion of the spinal canal near the dinosaur’s pelvis. The mysterious expansion is now thought to represent a glycogen body—a structure that stores energy-rich glucose and occurs in a similar position in some modern birds.
On supporting science journalism
If you’re enjoying this article, consider supporting our award-winning journalism by subscribing. By purchasing a subscription you are helping to ensure the future of impactful stories about the discoveries and ideas shaping our world today.
Present-day paleontologists remain unconvinced that Stegosaurus was capable of much higher reasoning. But in recent years scientists’ appraisal of the cognitive capacity of some other dinosaurs has improved, particularly that of members of the theropod lineage that gave rise to birds. With the advent of new technologies, such as micro computed tomography (CT) scanning, we can now reconstruct the volume and surface topography of brains without having to depend entirely on rare natural endocasts, greatly expanding the number of species available to study. Advanced imaging is also teaching us how dinosaurs might have used their brains. We now have the tools needed to answer the question of how long-vanished animals perceived the world around them and what really happened when predator met prey in the age of dinosaurs.
Where did T. rex fall on the intelligence spectrum between dim-witted Stegosaurus and tool-using ravens? In a high-profile paper published last fall, neuroscientist Suzana Herculano-Houzel of Vanderbilt University suggested that a T. rex was about as smart as a baboon—a startling conclusion because primates, with their large brains, are some of the cleverest animals around. Having spent long hours pondering the way brain volume scales with body size and what this relation means for brain function in extinct dinosaurs and birds, we were intrigued to see the headlines about this study. Superficially, the brain of the tyrant lizard king looks fairly puny compared with its body size. Weighing in at less than a pound, the brain of this six-ton dinosaur is diminutive next to the 11-pound brain of the African elephant, which, despite being the largest living terrestrial mammal has a smaller body than T. rex.
Herculano-Houzel argued that the relation between brain size and body size is unimportant when it comes to intelligence. What matters, she said, is the raw number of neurons in the telencephalon, a region in the front of the brain that includes not only the olfactory bulbs that process smell but also the cerebrum, where higher cognitive functions such as decision-making occur. Scientists previously had only an imprecise understanding of how many neurons were present in vertebrate brains because in different species they can be more or less densely packed in different parts of the brain.
A T. rex with the intelligence of a primate would be terrifying. We think some caveats are in order, however.
Herculano-Houzel and Roberto Lent of Federal University of Rio de Janeiro invented a technique for counting neurons called the isotropic fractionator method. It uses special chemicals to dissolve a brain, essentially making brain soup. A fluorescent dye stains the nuclei of neurons so that they glow and are easily visible. Researchers can precisely count the glowing nuclei in a small, homogeneous sample of the soup and then extrapolate the total number of neurons in the living brain. Using this method, Herculano-Houzel and her colleagues calculated that human brains have approximately 100 billion neurons, confirming earlier estimates.
The isotropic fractionator method is clever, but scientists will never have an actual T. rex brain to dissolve. Instead Herculano-Houzel relied on the scaling relation between telencephalon size and neuron numbers for living warm- and cold-blooded species, plugging extinct dinosaurs into one of two equations based on their inferred metabolism. This scaling relation varies tremendously among vertebrates. Cold-blooded, or ectothermic, species tend to have less tightly packed neurons than warm-blooded, or endothermic, species.
For example, isotropic fractionator data reveal that a 159-pound Nile crocodile has about 81.5 million neurons, whereas a 73-pound emu has roughly 1.3 billion neurons—almost 16 times as many as the crocodile despite being only half its size. Herculano-Houzel contended that most theropod dinosaurs were probably endothermic and thus near the bird end of the neuron-density continuum. Under this assumption, a T. rex telencephalon weighing roughly 12 ounces would contain around three billion neurons, on par with those of many primates.
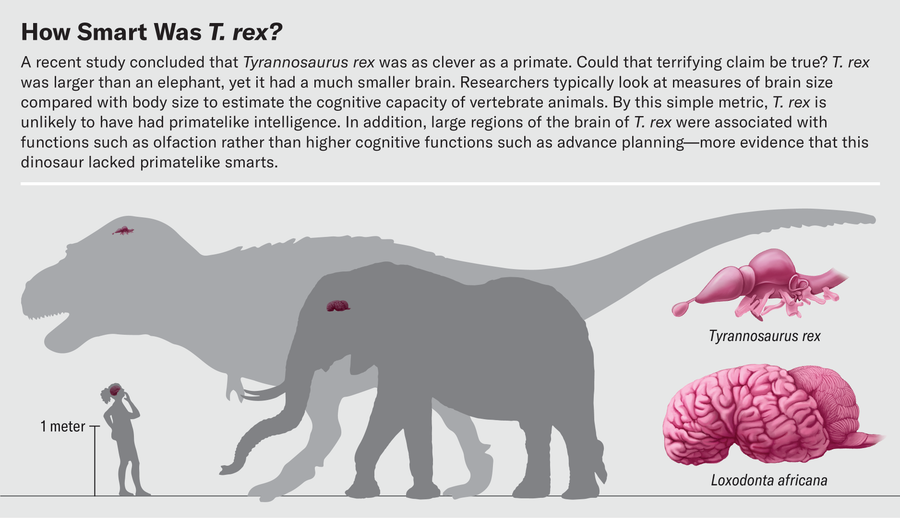
A T. rex with the intelligence of a primate would be terrifying. We think some caveats are in order, however. Besides the reasonable supposition that theropods were endothermic, the estimate of three billion neurons relies on a few other assumptions. One is that the entire braincase was occupied by the brain, which is clearly not true based on the morphology of T. rex endocasts. Fossil evidence indicates that in many dinosaurs, structures such as sinuses and blood vessels took up substantial parts of the braincase. Actual brain volume would have been smaller than raw endocranial volume. In fact, studies based on modern crocodilians suggest that the brain of T. rex might have occupied as little as 30 percent of the overall endocranial volume.
It’s also important to note that different brain regions have different functions. Some are devoted to tasks as basic as the regulation of breathing, whereas others support functions as complex as language. Two species with the same telencephalon size may have vastly different cognitive capacities if the cerebrum dominates the brain size of one and enlarged olfactory bulbs dominate the brain size of the other. For living species, it is possible to determine the boundaries between different brain regions with techniques such as cell staining or magnetic resonance imaging.
T. rex really would have been able to sniff the wind and identify both living prey and carcasses to scavenge long before laying eyes on them.
Defining these boundaries is much harder for dinosaurs because all we have to work from is the surface topology. One of us (Balanoff) has spent a significant part of her career mapping out bony landmarks that allow for better estimates of the volumes of major brain regions from endocasts. This work has shown that expansion of the cerebrum arose in more specialized theropods such as oviraptorosaurs and dromaeosaurs—lineages that branched off much later than tyrannosaurids. In contrast, earlier-diverging theropods such as T. rex had relatively small cerebrums, with a large portion of the overall telencephalon given over to the olfactory bulbs.
Once we account for the volume of nonneural tissues housed in the endocast, it’s unlikely that T. rex had three billion neurons of any kind in its telencephalon. We agree that T. rex was a proficient predator, but we argue that it was probably not capable of the advanced planning or coordinated social hunting seen in primates.
One of the best things about working with fossil endocasts constructed from CT scans is that we can study interior features without damaging the fossils themselves. Virtually chopping up fossil endocasts is a delightful way to spend an afternoon. Exploring the brain slice by slice, a trained paleontologist can use bony landmarks to decipher the boundaries of key brain regions and isolate those regions digitally. As we segment the brain from front to back, the olfactory bulb is the first structure we encounter. Olfactory bulb shape varies dramatically in dinosaurs and their relatives. Alligator olfactory bulbs are about the size of small grapes and are positioned at the end of long stalks leading to the rest of the brain. The olfactory bulbs of most birds are much smaller; in fact, in many species they are barely distinguishable from the rest of the cerebrum.
As the name implies, the olfactory bulb facilitates smell—a sense that relies on tiny molecules called odorants. Inhaled odorants bind to receptors in the nasal tissue, which communicate via neurons to the olfactory bulbs. Amazingly, each receptor makes a single odorant-receptor protein, which is tuned to specific types of odorants. Each of these proteins is coded by a different olfactory receptor gene. Genomic sequencing has revealed that birds have anywhere from 182 to 688 functional olfactory receptor genes.
In a recent study, Graham Hughes and John Finarelli of University College Dublin investigated dinosaurs’ sense of smell. The number of olfactory receptor genes in dinosaurs cannot be measured directly, but because bulb size correlates to the number of receptors, bulb dimensions can serve as a proxy for how well the animals could detect odors. Hughes and Finarelli found that, in general, dinosaurs had proportionally larger olfactory bulbs than birds. Among theropods, the omnivorous ornithomimosaurs had the smallest olfactory bulbs, and carnivorous species had the largest. Tyrannosaurus topped the charts with olfactory bulb dimensions consistent with the presence of more than 600 olfactory receptor genes. This number is on par with domestic cats and higher than in almost all modern birds. Our hypothetical Triceratops-stalking T. rex really would have been able to sniff the wind and identify both living prey and carcasses to scavenge long before laying eyes on them.
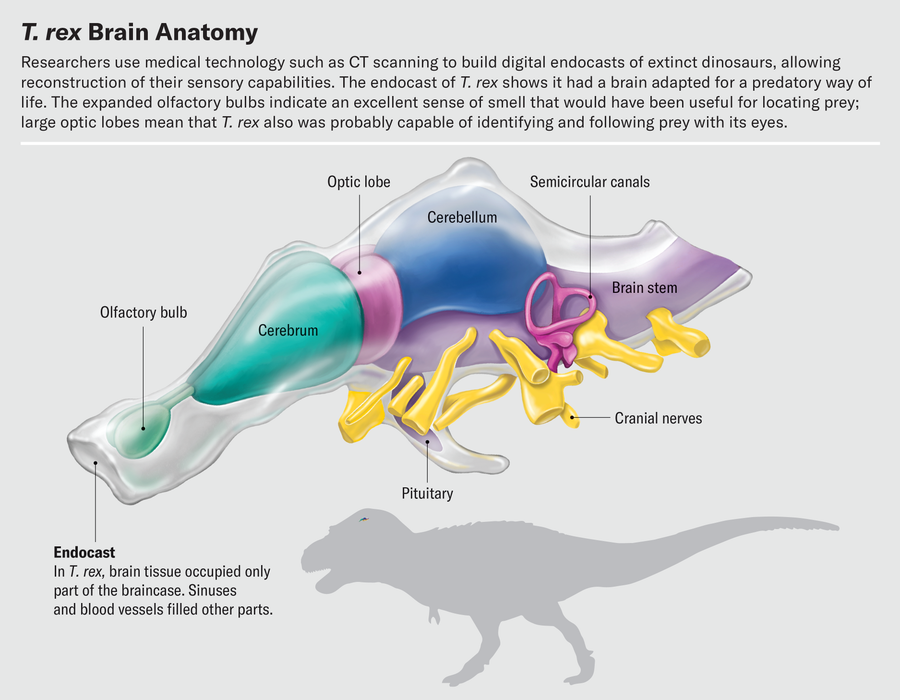
Mesa Schumacher; Sources: “New Insights into the Brain, Braincase, and Ear Region of Tyrannosaurs (Dinosauria, Theropoda), with Implications for Sensory Organization and Behavior,” by Lawrence Witmer and Ryan Ridgely, in The Anatomical Record, Vol. 292; 2009, and “Gross Anatomical Brain Region Approximation (GABRA): Assessing Brain Size, Structure, and Evolution in Extinct Archosaurs,” by Ashley Morhardt, Doctoral dissertation, Ohio University, 2016 (endocast references)
Other work on CT-based endocasts hints at how sharp T. rex’s eyes were. By virtually slicing up endocasts and isolating the optic lobes, we have found that the relative sizes of these structures were similar in extinct theropods and living birds. Birds therefore must have inherited their visual acuity from their nonbird ancestors. Birds are known to be highly visual animals—an eagle can spot a rabbit from half a mile away, and a tern in flight can track a fish half an inch long below the surface of water and pick it off from above. This reliance on sight is reflected in the structure of the bird brain. The optic lobes, which process visual information and reside just behind the cerebrum, are some of the brain’s most prominent features. It is often true that the larger a region is relative to the rest of the brain, the more important that region is to the animal. This certainly holds for the optic lobes.
We can infer some visual capacities of extinct dinosaurs from their evolutionary relationships. For example, both birds and crocodilians—the closest living relatives of the extinct dinosaurs—have the types of retinal receptors needed to see in color. So dinosaurs most likely had color vision, too. Vision is a complicated sense, however. Accurately reconstructing just how well extinct dinosaurs could see requires us to go beyond these types of inferences.
One factor that makes predatory birds so adept at capturing prey is stereoscopic vision—an enhanced ability to perceive depth. The sensory adaptation that underlies this sophisticated capability is astonishingly straightforward. It simply has to do with the position of the eyeballs. Animals with eyes positioned on the side of the head, such as geckos, lack overlapping visual fields, so they don’t see well in three dimensions. Animals with eyes positioned on the front of the head have visual fields that overlap in front of the nose. Within this overlapping space, each eye perceives the same information from a slightly different perspective, producing what is known as binocular vision.
Try fixing your gaze on an object about a foot or so in front of you, then closing one eye and then the other. The object will seem to move because you’re seeing the same image in front of your nose but from different angles. The brain integrates these slightly different images to produce visual depth. Animals with laterally positioned eyes judge depth by looking at something with one eye, moving their head and then looking at it with the other eye—not an especially stealthy technique. For a predator, binocular vision is particularly useful because it allows the animal to identify and zero in on prey without potentially disclosing its location by moving its head.
To determine whether extinct animals had stereoscopic vision, we must consider the position of their orbits, which are the spaces in the skull that house the eyeballs. Kent Stevens of the University of Oregon took a creative approach to this question by sculpting the heads of several theropod dinosaurs based on their skeletal structure. From there he was able to map their field of vision, including any obstructions such as horns or an especially large snout. He found that T. rex had forward-facing eyes and a narrow snout that wouldn’t obstruct its view, giving it a visual acuity similar to that of hawks. Unlike the fictional T. rex in Jurassic Park, a real T. rex would not have needed its prey to move to pick it out from the background. Deinonychosaurs such as Troodon and Velociraptor were probably even more adept at identifying their prey. With their heads slightly tilted forward, they had wider fields of vision and enhanced depth perception potentially rivaling that of owls.
We can infer more than the ability to perceive depth from the orbits of extinct animals. The size of these openings and their associated bones provide hints about the size of the eyeballs they housed. Larger orbits typically indicate larger eyeballs. Eyeballs that are large relative to the size of the head are common among nocturnal animals because they can accommodate larger numbers of light-sensitive photoreceptors in the retina. Moreover, the eyeballs of many animals, including fish, some extinct mammals, and reptiles (nonbird dinosaurs and birds among them), have bony or cartilaginous rings embedded within the fibrous outer layer of the eyeball known as the sclera. Because bone is rigid, these scleral rings constrain the movements of the eyeball, including how far the pupil can open. This affects how much light reaches the photoreceptors in the retina. Larger scleral rings allow the pupils to open wider, thereby letting more light into the eye.
A few years ago Balanoff was part of a team led by Jonah Choiniere of the University of the Witwatersrand in South Africa that studied the size of these rings in the orbits of nonbird dinosaurs to determine whether they preferred to move about during the daytime or at night. Because the scleral rings are not attached to any part of the skull, they are easily disassociated from the rest of the skeleton and rarely preserved in fossils. In the rare specimens that retain them, they are not always found in place. For this study, the researchers used CT images of extinct dinosaurs with preserved scleral rings to digitally isolate these bones and reconstruct them within the orbit. They concluded that the proportions of their orbits and scleral rings suggest many extinct dinosaurs were active primarily during the daytime.
But one house-cat-size alvarezsaurid theropod, Shuvuuia deserti, provided a different result. The team examined a Shuvuuia skull discovered in Late Cretaceous desert dune deposits from Mongolia and were startled to find adaptations convergent with one of the best-known living nocturnal animals, the barn owl. Barn owls have large orbits with ample scleral rings, which allow their pupils to open very wide. This arrangement lets an enormous amount of light flood into the eye, providing a picture in the darkest of conditions. The presence of these same features in alvarezsaurids suggests that they, too, were active nighttime predators.
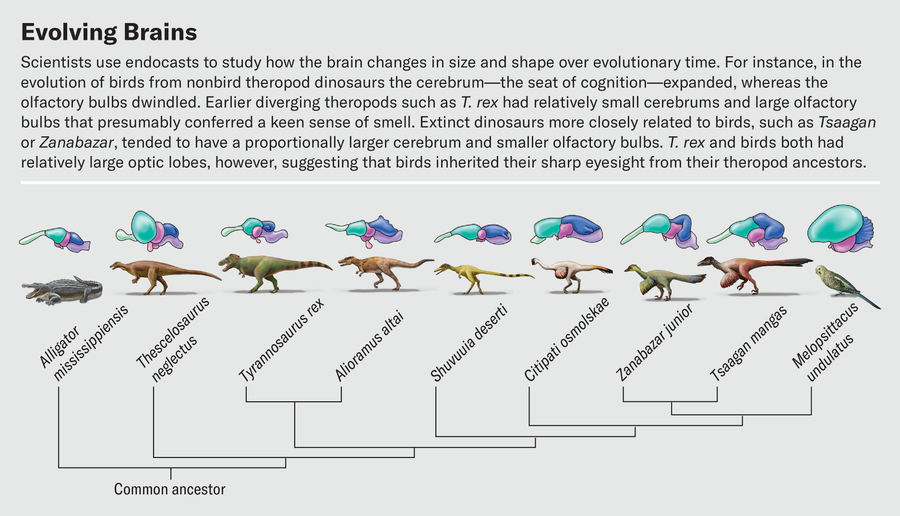
No one has formally analyzed the orbit morphology of T. rex for insights into when the dinosaur was most active. We do know, however, that T. rex had a large orbit shaped almost like a keyhole. If the eye had filled the entire orbit, then we might be able to infer that T. rex was nocturnal, but scleral rings from closely related species suggest that its eyeball filled only a small portion of the orbit—and thus might not have been able to gather enough light to be of much use at night. In fact, digital analyses of skull stresses led by Stephan Lautenschlager of the University of Birmingham in England indicate that the large orbit of T. rex helped to disperse the large stresses generated by its forceful bite rather than accommodating a big eye.
Near the rear of the vertebrate skull is an interesting and complex structure: the inner ear. Though not technically part of the brain, it is an important sensory organ that sends a lot of information to the brain. Two special senses, balance and hearing, are controlled by separate parts of the inner ear’s so-called labyrinth. The labyrinth comprises the semicircular canals, looplike structures that detect rotational movement of the head; the vestibule, a bloblike structure that senses back-and-forth and side-to-side movements; and the cochlea, which senses sound vibrations. The inner ear is filled with fluid and uses deflection of hair cells within that fluid to detect these different types of information.
In 2021 Michael Hanson, now at the Smithsonian Institution, and his colleagues carried out a sophisticated analysis of the shape of the semicircular canals and vestibule to infer the dominant mode of locomotion in nonbird dinosaurs. They made virtual endocasts of the inner ear space to estimate what the labyrinth would have looked like during life. Their data indicate that most dinosaurs were restricted to walking and running along the ground. But in the lineage that led to birds, the structure of the ear changed. Among other shifts, the semicircular canals grew longer. This elongation allowed dinosaurs to make and interpret more complex movements of the head. T. rex didn’t have long semicircular canals, suggesting that it was capable of only walking or running. But some troodontid dinosaurs more closely related to Velociraptor had an ear that could sense the complex movements associated with flight. These dinosaurs were probably capable of gliding or a rudimentary form of flight, moving through the air before modern birds took wing.
We can look to the cochlea for clues to dinosaurs’ hearing. The length of the cochlea is correlated with hearing sensitivity. A longer cochlear duct allows for an elongation of the basilar papilla, the structure that holds the hair cells that pick up sound vibrations. Lizards and turtles tend to have short, stubby cochleas best suited to detecting low-pitch sounds. Crocodilians and birds, in contrast, have much longer, slenderer cochleas that excel at detecting higher-pitched sounds.
Many birds sing melodious songs to attract mates and defend their territory, so it might seem fitting that they have increased sensitivity to high-pitched vocalizations. Yet the elongation of the cochlea originated not in birds but in the common ancestor of birds and crocodilians. The weird thing is, croc vocalizations are limited to low-pitched, closed-mouth grunts—not the kinds of sounds that an elongated cochlea excels at detecting. Exactly why crocodilians have such an advanced cochlea shape compared with other reptiles was a mystery.
Hanson and his collaborators proposed a clever explanation. They hypothesized that the elongation of the cochlea that differentiates crocodilians, extinct dinosaurs and birds from earlier-diverging reptiles has to do with the evolution of parental care. Unlike most reptiles, crocodilians care for their young. And unlike most young reptiles, crocodilian babies chirp to get their parents’ attention. Perhaps the ancestors of birds and crocs needed to be able to detect high-pitched sounds to hear their young rather than mates or rivals.
Like modern predators, T. rex had a proportionally large brain compared with its plant-eating quarry.
This hypothesis has exciting implications for the evolution of birdsong. In this scenario, juvenile dinosaurs could have chirped for attention, but adults were restricted to producing simple low-pitched calls. Over the course of the dinosaur-bird transition, some lineages retained the capacity for high-pitched vocalizations into adulthood. Cochlear elongation, originally favored by natural selection for its role in enhancing parental care, then served as a preadaptation that allowed song to arise in later birds.
But evolution doesn’t move in a single direction. Sifting through the dinosaur data, Hanson and his colleagues stumbled on an unusually short cochlea in Alioramus, a cousin of Tyrannosaurus. This discovery suggested that the large theropod had lost sensitivity to higher-pitched sounds. The team speculated that Alioramus might have left its young unattended, removing the selection pressure for hearing their vocalizations.
The shape of the cochlea has also helped us understand the ecology of those oddball nocturnal alvarezsaurids. Balanoff and her colleagues found that the cochlea of Shuvuuia was so long that it curled under the base of the skull. Nocturnal birds such as owls are known to have a similar arrangement. The hyperelongated cochlear duct of Shuvuuia indicates that its hearing was attuned to very high-frequency sounds such as those produced by insects. The team concluded that this small dinosaur prowled the desert dunes of Central Asia in the darkness, hunting for these small prey.
People tend to think of paleontology as a field-based discipline, focusing on the romantic allure of summers spent in remote desert locales with pickax in hand, collecting fossils of long-extinct animals new to science. But these days paleontologists are just as likely to make their most significant discoveries in the laboratory using cutting-edge technologies from biomedicine and neuroscience. It is the combination of these disparate approaches that allows us to reconstruct what really might have gone down when T. rex encountered Triceratops.
Our own research tells us that like modern predators, T. rex had a proportionally large brain compared with its plant-eating quarry. A substantial part of its brain was devoted to olfaction, so Tyrannosaurus probably did sniff the air to locate its next meal, whether it was the living Triceratops grazing along the tree line or one that was already dead and rotting in the sun. Once the T. rex isolated a scent, it could then scan the horizon with its stereoscopic vision for any sign of potential prey. Its eyes would have been able to fix on that Triceratops obliviously feeding on a cluster of vegetation far from the safety of its herd.
As the T. rex crashed through the trees, it might have startled a small troodontid dinosaur nesting in the branches nearby. With the enhanced locomotor skills afforded by its expanded inner ear labyrinth, the troodontid might have glided off its nest, distracting the predator from its chirping young. Unsettled by this commotion, the Triceratops might have stopped its peaceful grazing and returned to the safety of its herd. It’s still a clichéd story but one that is much more scientifically informed.